The complete guide to learning the krebs cycle in med school
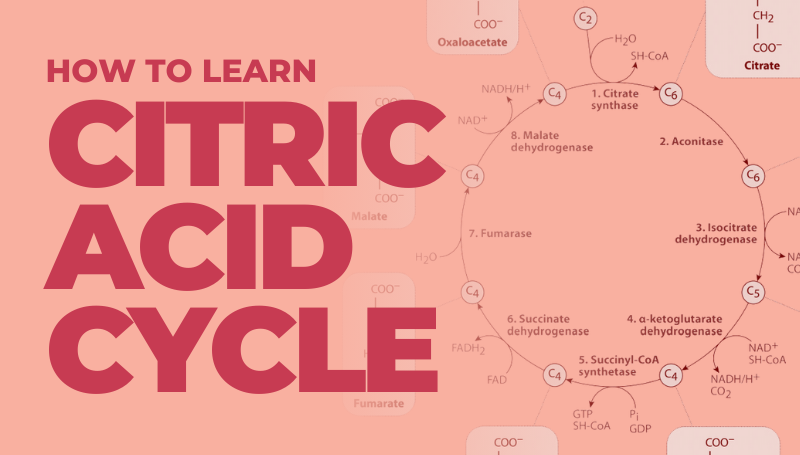
The Krebs cycle—also known as the citric acid cycle—is a critical component of medical education. As a foundational concept in biochemistry, it plays a pivotal role in understanding how cells generate energy, which is essential for everything from muscle contraction to brain function.
Many medical students find the Krebs cycle challenging due to its complexity and the sheer volume of details involved. The cycle's intricate series of chemical reactions can be daunting, but mastering it is crucial for success in both medical school and future clinical practice. Try to break down the cycle into smaller, more manageable parts, using visual aids like diagrams and flowcharts. You can also test yourself with practice questions to enhance your understanding.
The Medical Student Core: Biochemistry provides medical students with a comprehensive look at the citric acid cycle, and includes everything you need to learn about it during your med school training.
The content below is excerpted from the Medical Student Core > Biochemistry > Citric Acid Cycle. This MedStudy Core content was medically reviewed by Tony Hannaman, MD.
Before you start studying the Kreb's Cycle
Before you continue reading, pause and try to answer these Preview | Review Questions. These will prime your brain for the material. Try to think of everything you already know about the Citric Acid Cycle in order to answer.
This helps you brain dredge up any older memories that exist so it an re-encode them as you learn. It also primes your brain to understand what will be important in the upcoming information. You'll find the answers to each question highlighted in the content below.
There are Preview | Review Questions like this for almost every topic in the Medical Student Core. They cover the most important information on the topic—pertinent to your training as a future doctor, as well as licensing exams.
Krebs cycle Preview | Review questions from the Medical Student Core
Citric Acid Cycle — Overview
The citric acid cycle (a.k.a. tricarboxylic acid [TCA] cycle or Krebs cycle, for Hans Adolph Krebs who identified it in 1937) is a series of biochemical reactions used by all aerobic organisms to produce energy from carbohydrates, protein, and fat. This occurs through the oxidation of acetyl-CoA, leading to ATP production. In contrast to glycolysis, the citric acid cycle occurs in mitochondria.
This process was named the citric acid cycle because it begins with conversion of acetyl-CoA to citric acid (a.k.a. citrate). The citrate is consumed and eventually regenerated to continue the cycle. Citrate is a type of TCA.
Think of the citric acid cycle as the biochemical crossroads of the cell. First, it is the final common pathway for the oxidation of fuel, meaning carbohydrates, amino acids, and fatty acids. Any molecule that can be broken down into an acetyl group or dicarboxylic acid can enter this pathway to produce ATP. Recall that glycolysis produces only a small amount of ATP from glucose. Further processing in the citric acid cycle (in conjunction with oxidative phosphorylation) is the main source of ATP generated in metabolism, accounting for about 95% of energy in aerobic cells. Second, there are many important byproducts of the reactions in the citric acid cycle. These include precursors for amino acids, nucleotide bases, cholesterol, and porphyrin, as well as NADH and other forms of energy.
Figure 19: NADH and FADH2 production in the citric acid cycle
The cycle is a series of 8 enzymatic oxidation-reduction, or redox, reactions. Oxidation is a chemical reaction in which a molecule loses electrons, whereas reduction is a chemical reaction in which a molecule gains electrons. Redox reactions involve the transfer of electrons between molecules during the same reaction. Chemically, the citric acid cycle produces high-energy electrons from carbon fuel (Figure 19). It does this by removing electrons from acetyl-CoA and using them to generate NADH and the reduced form of flavin adenine dinucleotide (FADH2 ) (Figure 20). NADH and FADH2 transfer their electrons in the electron transport chain, and through oxidative phosphorylation, generate most of the ATP in aerobic cells. Oxygen is not used directly in the citric acid cycle. It is the final electron acceptor at the end of the electron transport chain, regenerating NAD+ and FAD.
Figure 20: Electron production in the citric acid cycle and use in oxidative phosphorylation
Substrate for the Citric Acid Cycle
Once acetyl-CoA is in a mitochondrion, it cannot be transported out. There are 2 main sources of acetyl-CoA:
-
- Glucose: At the end of glycolysis, the PDC converts pyruvate to acetyl-CoA.
- Fatty acids: β-oxidation of fatty acids yields acetyl-CoA. Note that β-oxidation of some fatty acids produces propionyl-CoA, which is converted to succinyl-CoA. Succinyl-CoA can enter the citric acid cycle as an intermediate.
Did you notice that we did not mention proteins? So, how do proteins enter the citric acid cycle? Proteases break proteins down into their constituent amino acids. Their carbon skeletons can enter the citric acid cycle in a few different ways:
-
- By conversion into intermediates; for example, glutamine is processed to form α-ketoglutarate.
- By conversion into acetyl-CoA; this is possible for several amino acids, including leucine, isoleucine, lysine, phenylalanine, tryptophan, and tyrosine.
- By conversion into pyruvate; this is possible for alanine, cysteine, glycine, serine, and threonine.
Steps of the Citric Acid Cycle
Note Figure 21 as you review the 8 reactions of the citric acid cycle.
Figure 21: The citric acid cycle
8 Steps of the Citric Acid Cycle:
-
- A 2-carbon unit of acetyl-CoA condenses with a 4-carbon unit of oxaloacetate to form a 6-carbon unit of citrate. ◦ Citrate synthase catalyzes this reaction.
- Aconitase catalyzes the isomerization of citrate to isocitrate. ◦ Isocitrate is much more readily oxidized than citrate. •
- Isocitrate dehydrogenase catalyzes the oxidative decarboxylation of isocitrate. This removes a unit of CO2 , resulting in a 5-carbon unit of α-ketoglutarate. ◦ This reaction requires the input of NAD+ and produces NADH.
- The α-ketoglutarate dehydrogenase complex catalyzes the oxidative decarboxylation of α-ketoglutarate. This removes another unit of CO2 , resulting in a 4-carbon unit of succinyl-CoA, which is an energy-rich compound. ◦ This reaction also requires the input of NAD+ and produces NADH.
- Succinyl-CoA synthetase cleaves succinyl-CoA to produce succinate. ◦ This reaction is coupled with phosphorylation of guanosine diphosphate (GDP) to produce guanosine triphosphate (GTP). (Note that it is possible to convert GTP to ATP. The enzyme nucleoside diphosphokinase catalyzes the reaction of GTP + ADP → GDP + ATP.)
- Succinate dehydrogenase oxidizes succinate to fumarate. ◦ This is a difficult oxidation reaction, so it utilizes FAD, which is a more powerful oxidant than NAD+ . The FAD is reduced to FADH2.
- Fumarase hydrates (adds H2 O) fumarate to produce malate.
- Malate dehydrogenase oxidizes malate to produce oxaloacetate. ◦ This reaction requires the input of NAD+ and produces NADH.
Citric Acid Cycle Intermediates
Some of the intermediates formed in the citric acid cycle leave the cycle to enter other biosynthetic pathways. Although acetyl-CoA cannot leave the mitochondrion, citrate can. Once it is in the cytoplasm, the citrate can be converted back into acetyl-CoA for use in fatty acid and cholesterol synthesis. Likewise, malate can leave the mitochondrion. In the cytosol, malate is oxidized back to oxaloacetate, which enters the gluconeogenesis pathway.
Some intermediates provide the carbon skeletons for nonessential amino acids (i.e., amino acids the body can make, which means they do not have to be consumed in the diet). α-ketoglutarate is processed to form glutamine, proline, and arginine. Oxaloacetate is processed to form aspartate and asparagine.
Succinyl-CoA provides the carbon needed for synthesis of porphyrins, which are heterocyclic organic compounds. Porphyrins are important components of hemoglobin, myoglobin, and cytochromes.
Citric acid cycle intermediates are depleted in the reactions involving synthesis of fatty acids, cholesterol, glucose, nonessential amino acids, and porphyrins. Other processes that replenish the supply of intermediates of metabolic pathways are referred to as anaplerotic pathways. The flow of intermediates into and out of the citric acid cycle is balanced, so that concentrations of these molecules within the mitochondria remain constant over time.
Summary of Energy Generation
In a single turn of the citric acid cycle:
-
- 2 carbon atoms enter the cycle in the form of acetyl-CoA, and 2 molecules of CO2 are released.
- 3 NADH and 1 FADH2 are generated.
- 1 GTP is produced, which ultimately is converted to ATP.
The chemical equation representing the sum of the 8 reactions in a single turn of the citric acid cycle is:
Acetyl-CoA + 2 H2O + 3 NAD+ + FAD + GDP + Pi →
2 CO2 + 3 NADH + 3H+ + FADH2 + uncombined coenzyme A (CoASH) + GTP
Note that 1 molecule of glucose yields 2 molecules of acetyl-CoA, so you will actually want to double the above numbers to show the yield for each molecule of glucose. So, for 1 glucose molecule, the energy output for the citric acid cycle is 2 ATP, 6 NADH, and 2 FADH2 . You can see that at this point we have not generated that much more ATP than we did in glycolysis. But look at all the energy-rich NADH and FADH2 we generated! The big payoff arrives in the electron transport chain, which generates about 3 ATP per NADH oxidized and 2 ATP per FADH2 oxidized. In total, aerobic cellular respiration yields approximately 38 ATP from the oxidation of 1 molecule of glucose!
Key takeaways for learning the Kreb's Cycle in medical school
- The Krebs Cycle is an intricate series of chemical reactions—it can be daunting to learn, but mastering it is crucial for success in both medical school and future clinical practice. Try to break down the cycle into smaller, more manageable parts, using visual aids like diagrams and flowcharts. You can also test yourself with practice questions to enhance your understanding.
- When it comes to the Kreb's Cycle, you'll likely see test questions on: what it is, where it occurs, the reaction types, and what it accomplishes.
- The Citric Acid Cycle is a series of biochemical reactions used by all aerobic organisms to produce energy from carbohydrates, protein, and fat. This occurs through the oxidation of acetyl-CoA, leading to ATP production. In contrast to glycolysis, the citric acid cycle occurs in mitochondria.
- There are 2 main sources of acetyl-CoA: Glucose and Fatty acids.
- For 1 glucose molecule, the energy output for the citric acid cycle is 2 ATP, 6 NADH, and 2 FADH2
Mastering the Krebs cycle is no small feat, but it’s an essential milestone on your path to becoming a successful physician. While the intricacies of this cycle may seem overwhelming at first, break it down into smaller components, use visual aids, and keep studying! Remember, every step of the cycle you understand brings you closer to grasping the larger picture of cellular metabolism and energy production.
Did you love the conversational language in this Core excerpt blog? Our Medical Student Core offers clear explanations, visual aids, and practical tips to help you conquer the Krebs cycle and other challenging concepts in medical school and on USMLE exams.